Content from 2016-04
Tags
Months
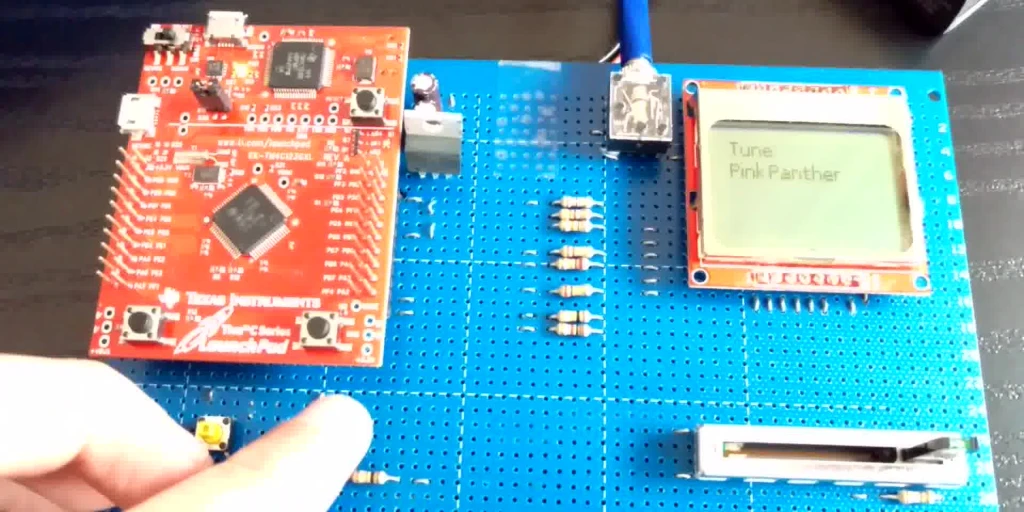
It would not be a cool game if it did not make funky noises. This post describes a way to build a sound device using a DAC and a bunch of resistors. This device will be able to play Nokia's RTTTL tunes.
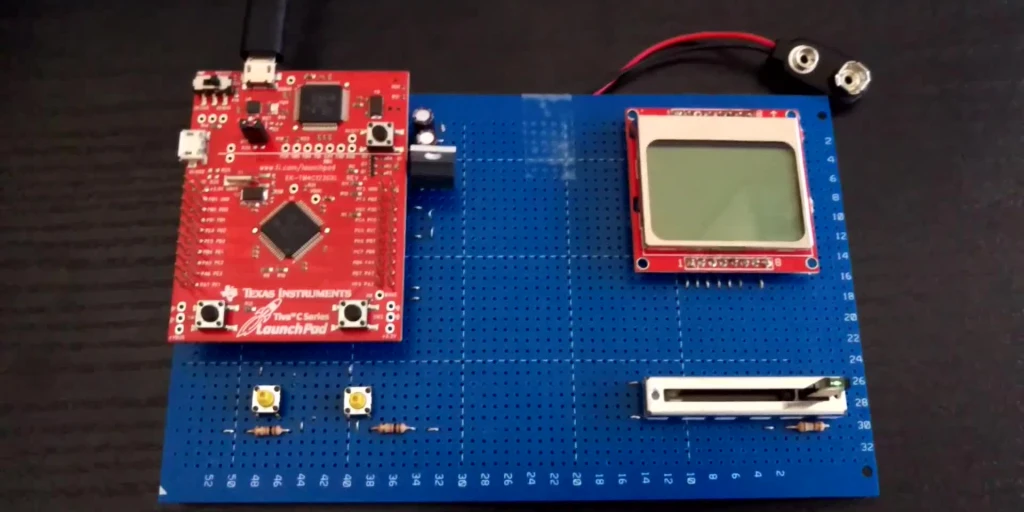
We need to handle IO to move the defender and fire at the invaders. GPIO-connected buttons will do fine to trigger the shots, and we will read out a potentiometer through an ADC to determine the defender's position. This post describes the HAL functions for that and for handling timers, which is always helpful.
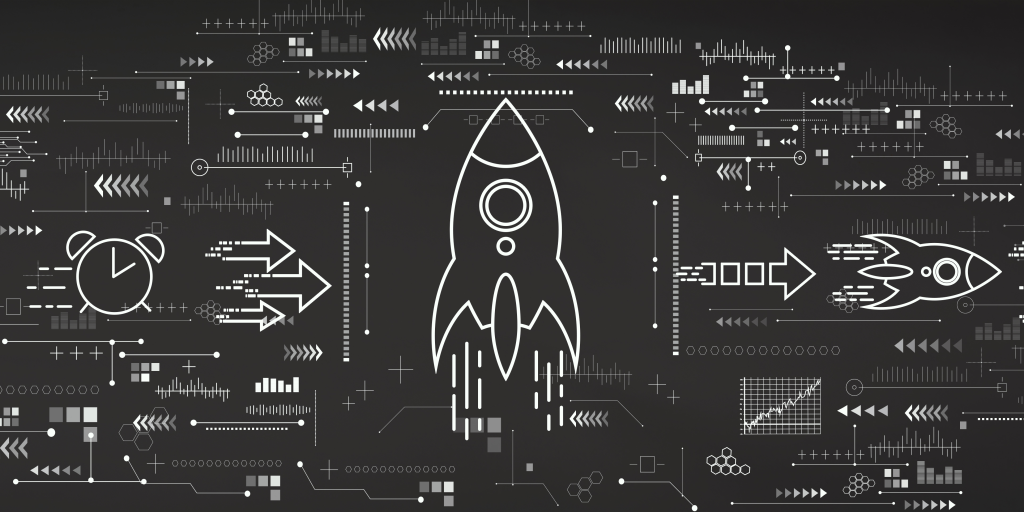
Paper summary: The Linux Scheduler: a Decade of Wasted Cores by J.-P. Lozi et al. The authors found issues with the Completely Fair Scheduler on a NUMA system with 64 cores that kept cores idle while tasks were waiting to be executed. Fixing these problems resulted in a 138 times speedup in an extreme case.
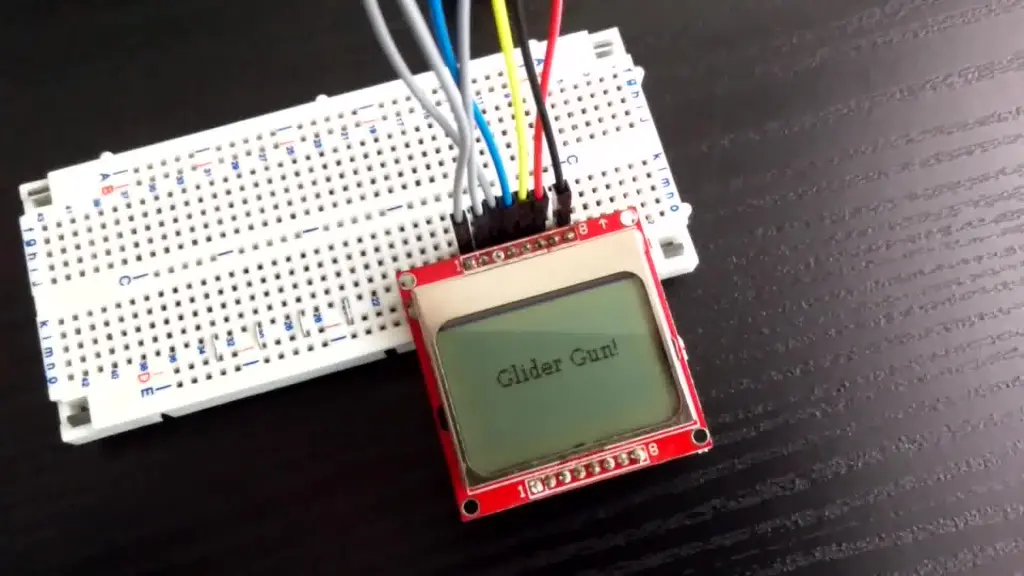
It's obvious, but Silly Invaders needs a display to display invaders. This post describes HAL calls to handle an old Nokia phone display through SSI and other things that were necessary to make it work, such as heap management.
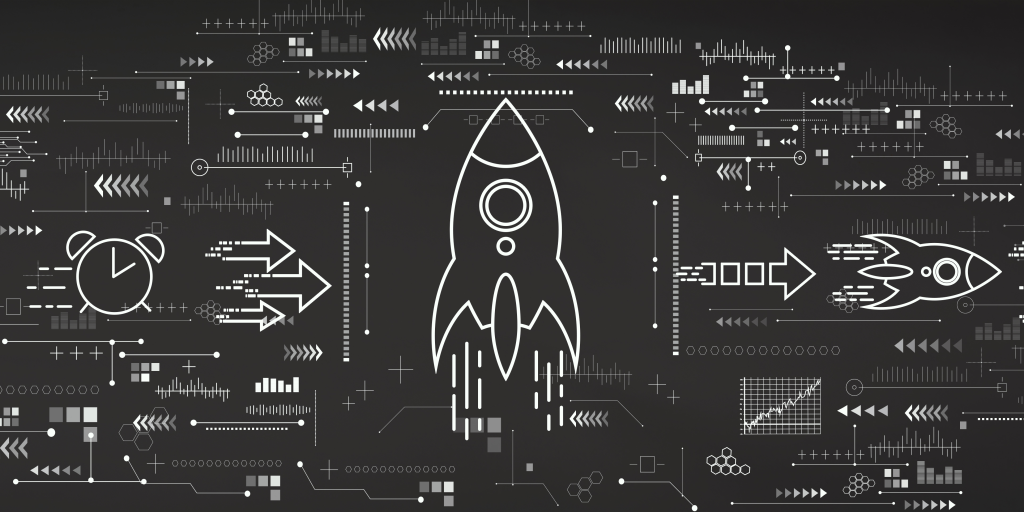
I want my Alien Invaders to be portable and work on other microcontrollers. For that reason, the game logic will use a Hardware Abstraction Layer to communicate with the device. This post describes a stem of that layer dealing with hardware initialization and UART. Every future post will extend it with new functionality.